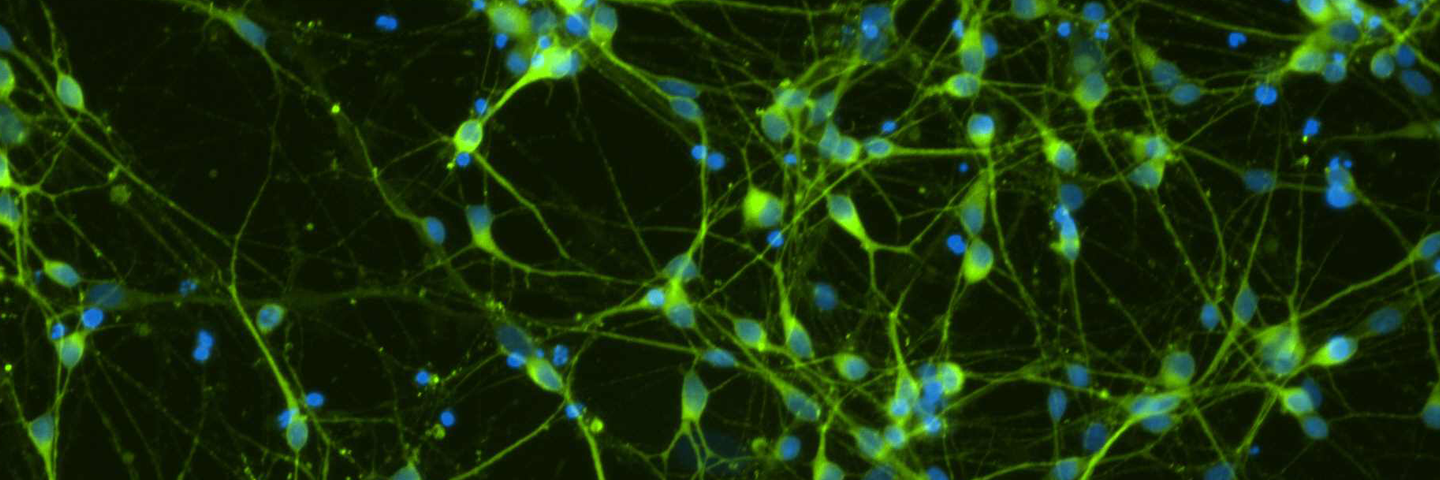
Authors: Leelamma M. Panicker, PhD; Michelle Spencer, MS; Brian Shapiro, PhD; and Dezhong Yin, PhD
Introduction
Materials and Methods
Results/Discussion
Conclusions
References
Abstract
Neurological models are critical to understanding the physiology of the nervous system and for assessing the neurotoxicity of pharmaceuticals and environmental compounds. In this study, we examined the ability of a novel medium to stimulate neural progenitor cells (NPCs) to differentiate into multiple types of neurons. To determine the suitability of the NPC culture system for use in toxicological studies, we investigated the sensitivity of the NPCs and dopaminergic neurons for various known toxic compounds.
Download a PDF of this application note
Download NowIntroduction
Human induced pluripotent stem cell (iPSC)-derived neural progenitor cells (NPCs) and neurons are an attractive in vitro model to study neurological development, neurotoxicity, and to model disease. However, there is a lack of validated NPC lines and media that support differentiation into multiple types of neurons for disease modeling, drug screening, and toxicity screening.1-6
New model systems that recapitulate the components of the nervous system are vital for novel neurobiological discoveries and for toxicological testing. Because the nervous system is one of the most complex organ systems, it is difficult to recreate in a culture dish. Neurons do not expand quickly or at all, neural differentiation occurs over months, and knowing when your neurons are ready for experiments can be challenging. Current in vitro models of the nervous system typically consist of tissue slices or neurons isolated from cadavers and animals, neural cells derived from continuous cell lines (eg, SH-SY5Y, Neuro-2a, and PC-12), and neural cells derived from iPSCs.7-12 However, primary sources can suffer from donor variation and continuous cell lines may not faithfully represent the in vivo state. Additionally, it can take two or more months before iPSC-derived differentiated neurons are ready for experimentation.13
NPCs combine the best qualities of continuous cell line–, primary cell–, and iPSC-derived neurological models. NPCs can be expanded over 10 passages and fully differentiate into neurons in as little as 3 weeks. In addition, NPCs do not suffer from donor variation because they are derived from a single clone. ATCC recently created a complete NPC system of cells and media supplements. This application note describes the characterization and validation of 2 of those cell lines, an expansion medium, and a dopaminergic neuron differentiation medium.
Materials and methods
For dopaminergic neuron differentiation, a normal human non-reporter NPC line (ATCC ACS-5003) and a MAP2- NanoLuc HaloTag reporter NPC line (ATCC ACS-5007) were seeded in ATCC Cell Basement Membrane (ATCC ACS-3035)-coated 12-well plates at a seeding density of 10,000 cells/cm2 and cultured in NPC expansion media (ATCC ACS-3003) overnight prior to treating NPCs with Dopaminergic Differentiation Media (ATCC ACS-3004) for up to 3 weeks. For qRT-PCR, RNA was extracted from ACS-5003 and ACS-5007 NPCs treated with the dopaminergic differentiation media for 0, 1, 2, or 3 weeks and cDNA was synthesized for qRT-PCR analysis of neuronal gene expression by using TaqMan probes (ThermoFisher Scientific, Inc.) shown in Table 1. For neurotoxicity assays, the ACS-5003 and ACS-5007 NPCs were seeded in Cell Basement Membrane-coated 96-well plates at a seeding density of 40,000 cells/cm2 and cultured in NPC expansion media for 2 days prior to adding multiple neurotoxicants at 1 μM, 10 μM, or 100 μM for 2 days. Neurotoxicity was assessed by using resazurin viability dye. To examine neurotoxicity of neurons using resazurin and high-content imaging methods, ACS-5003 and ACS-5007 NPCs were seeded in Cell Basement Membrane-coated 96-well and 24-well plates, respectively, at a seeding density of 10,000 cells/cm2 and treated with dopaminergic differentiation media for 3 weeks prior to adding various concentrations of drugs for 2 days.
Table 1. TaqMan probes used for gene expression analysis
Type of neurons | Name of TaqMan probes |
---|---|
Early neurons | MAP2, TUBB3 (Tuj1) |
Dopaminergic neurons | TH, Nurr1, VMAT2, DAT, AADC |
Glutamatergic neurons | GLS2, vGLUT1, vGULT2 |
GABAergic neurons | GABRB3 (GABA) |
Motor neurons | EN1, LIM3, HB9 |
Cholinergic neurons | ChaT |
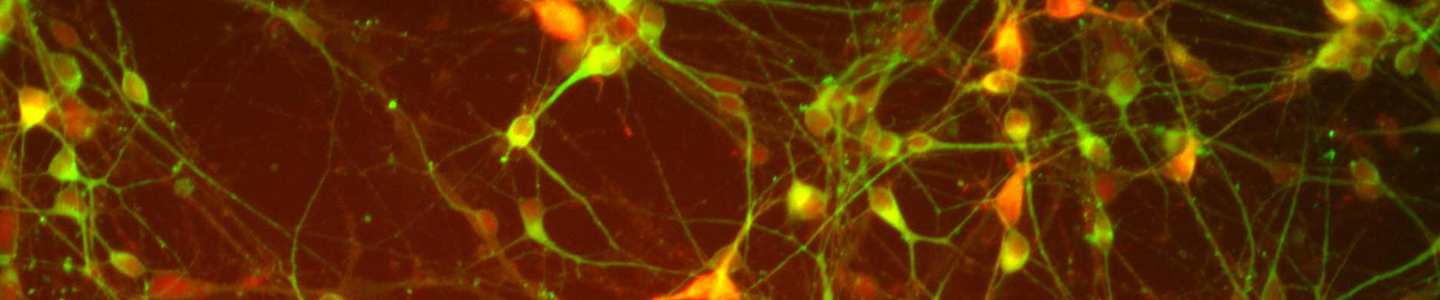
Results/Discussion
Here, we investigated the expression of genes associated with the differentiation of NPCs during 3 weeks in dopaminergic differentiation media. Known early neuron markers MAP2 and Tuj1 genes reached peak expression at 2 weeks while expression of dopaminergic neuronal genes (TH, Nurr1, VMAT2, AADC) was significantly increased in a time-dependent manner (p < 0.05) in 2 types of normal NPCs (Figure 1). Furthermore, expression of genes associated with glutamatergic (vGLUT1, vGLUT2, GLS2; Figure 2) and GABAergic (GABRB3; Figure 3) neurons was also induced and peaked at the end of 3 weeks. This shows that ATCC NPCs and dopaminergic differentiation media are capable of producing GABAergic and glutamatergic neurons in addition to dopaminergic neurons. Noticeably, there was significant induction of motor neuron gene (EN1, LIM3, Hb9) expression in ACS-5003 NPCs while there was significant induction of cholinergic (ChAT) neuron gene expression in ACS-5007 NPCs but not in ACS-5003 NPCs during dopaminergic differentiation (Figure 3). Moreover, expression of TH, Tuj1, Nurr1, GLS2, and vGLUT2 neuronal markers in both ACS-5003- and ACS-5007-derived neurons has been confirmed by immunocytochemistry (Figure 4). To validate that ATCC NPCs and dopaminergic neuron differentiation media are suitable for drug screening, we conducted neurotoxicity screenings in the two types of NPCs and NPC-derived neurons by using a resazurin viability assay and high-content imaging analysis. We found that paclitaxel, a microtubule-stabilizing chemotherapeutic agent, significantly induced neurotoxicity (p < 0.001) in the two types of NPCs evaluated at a IC50 of ~ 1 μM, but not in NPC-derived neurons (Figure 5 and Figure 6). Vincristine, amiodarone, and chlorhexidine significantly decreased viability of both NPCs and neurons, whereas piperine, cisplatin, and hydroxyurea did not induce any significant neurotoxicity in neurons (Figure 5 and Figure 7). This study demonstrates that ATCC iPSC-derived NPCs and dopaminergic differentiation media are suitable for studying neurological development and neurotoxicity screening.
Conclusions
Human iPSC-derived NPCs and neurons provide an unlimited resource for in vitro disease modeling, toxicity screening, and drug screening. ATCC has developed normal and lineage-specific GFP or Nanoluc-HaloTag reporter NPCs. The normal and reporter-labeled NPCs have the potential to be differentiated into dopaminergic, GABAergic, glutamatergic, motor, and cholinergic neurons after treatment with ATCC Dopaminergic Differentiation Media. In addition, NPCs and NPC-derived neurons have been validated for drug screening with several neurotoxicants by using a resazurin viability assay and high-content imaging assessment. Therefore, ATCC NPCs, NPC Expansion Media, and Dopaminergic Differentiation Media are suitable for studying neurological development and neurotoxicity screening.
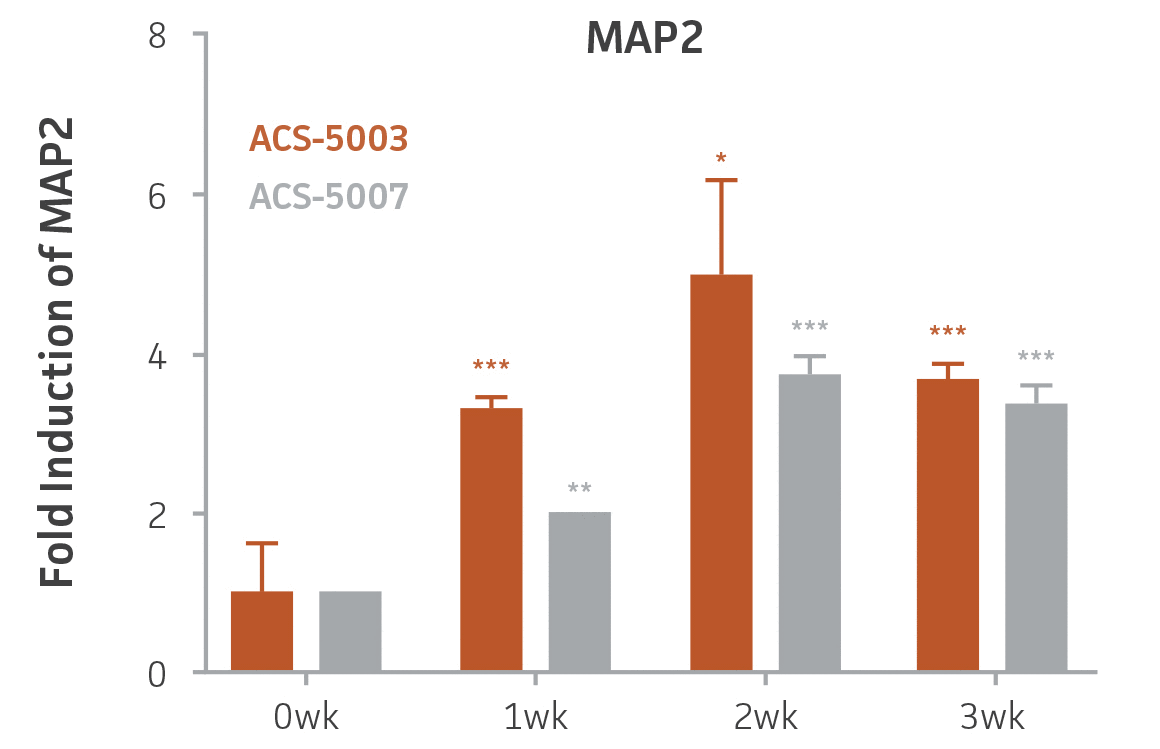
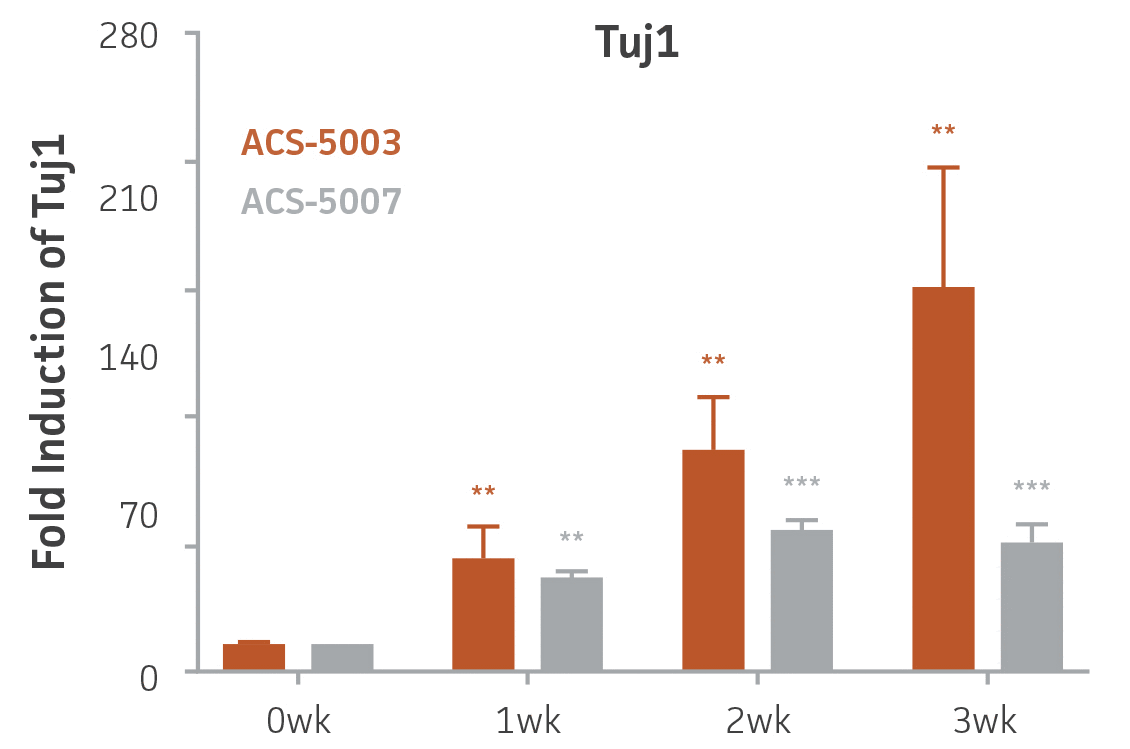
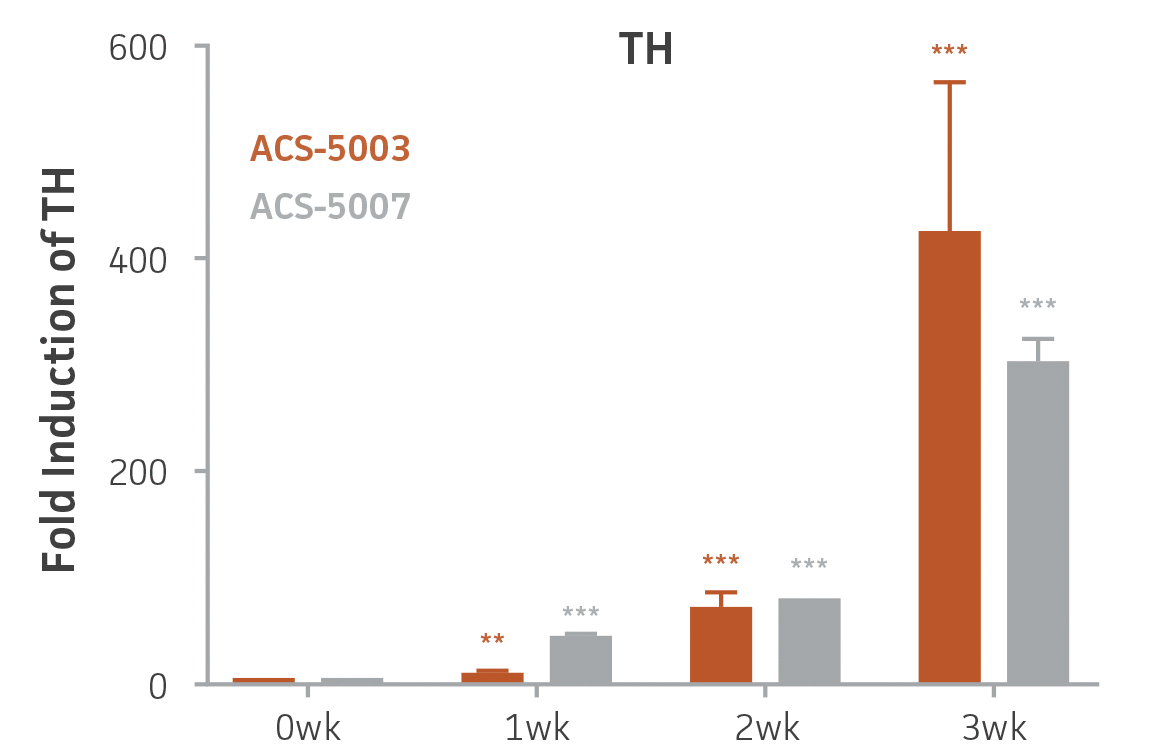
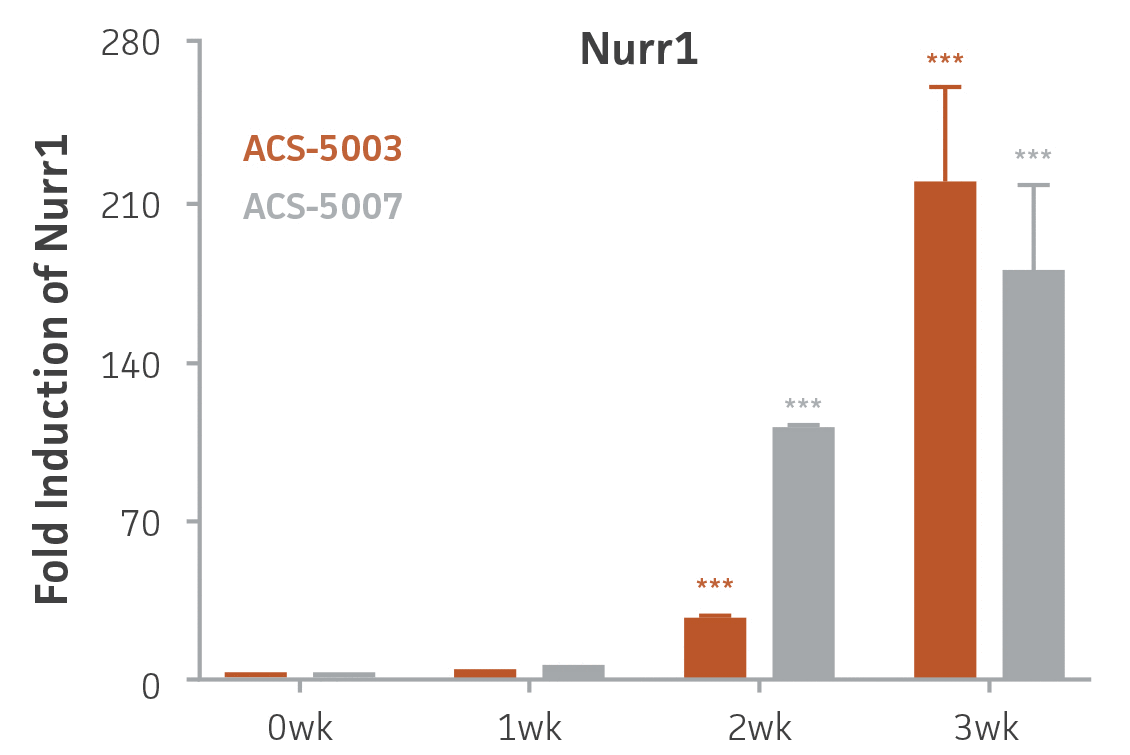
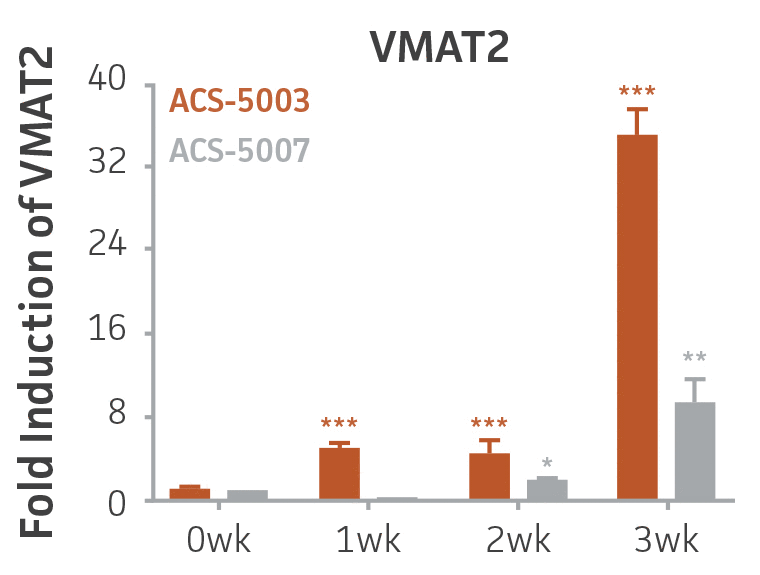
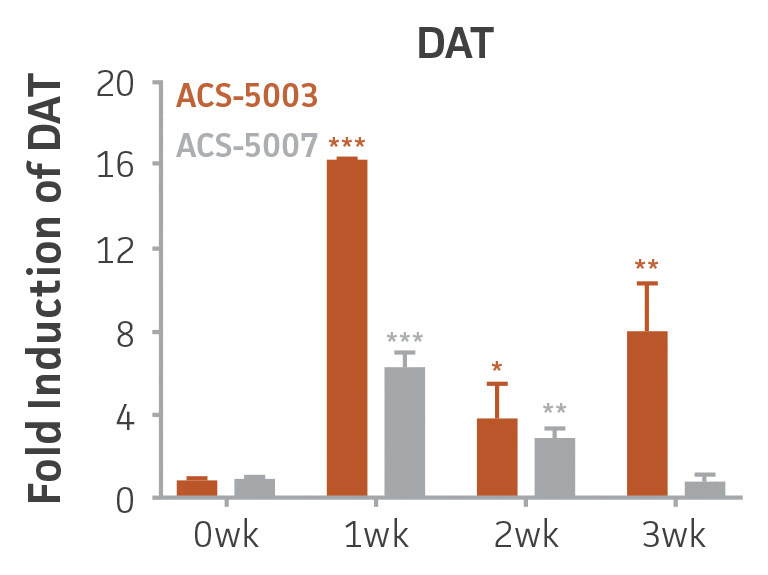
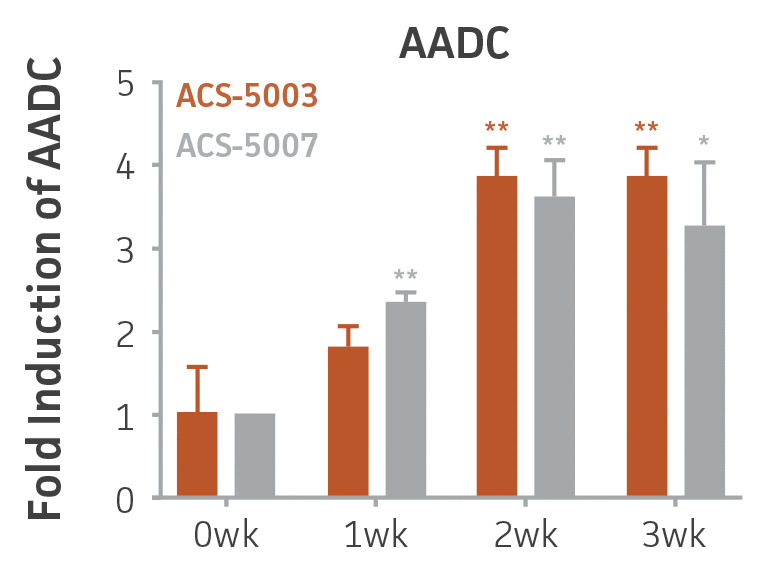
Figure 1. Induction of neuronal gene expression during 3 weeks of dopaminergic neuron differentiation. Expression of early neuronal genes (MAP2 and Tuj1) increased significantly in both ACS-5003 and ACS-5007 within a week (wk) of dopaminergic media treatment. In contrast, TH and dopaminergic neuronal transcription factor Nurr1 mRNA increased significantly and reached maximum level by the end of 3 weeks of differentiation. Related genes like VMAT2 and DAT with opposite functions also increased significantly. AADC also significantly increased in a time-dependent manner in ACS-5003 and ACS-5007 during neuronal differentiation (n=1, *p < 0.05, **p < 0.01, ***p < 0.001 vs. day 0, Two-Way ANOVA).
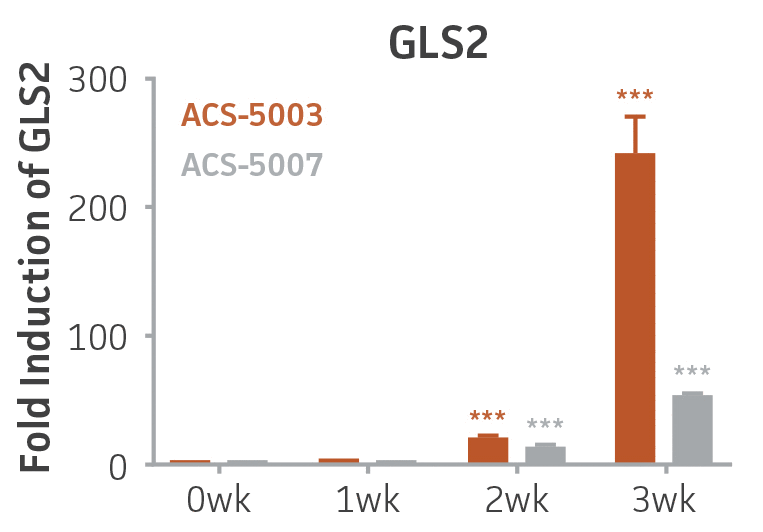
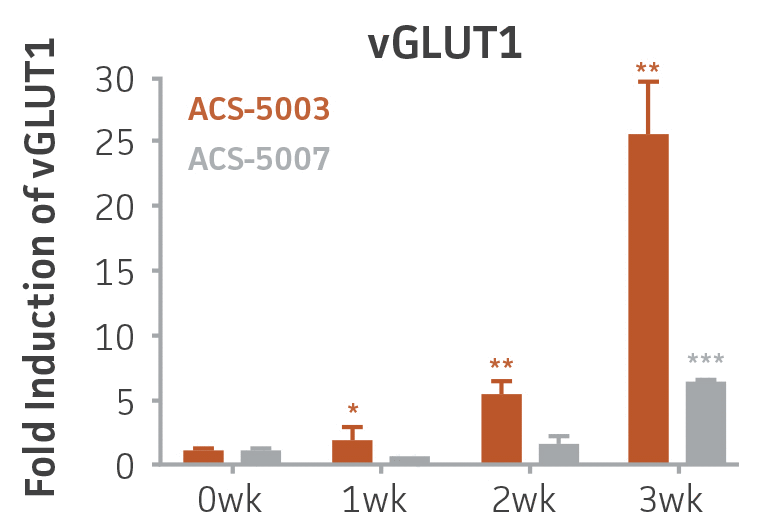
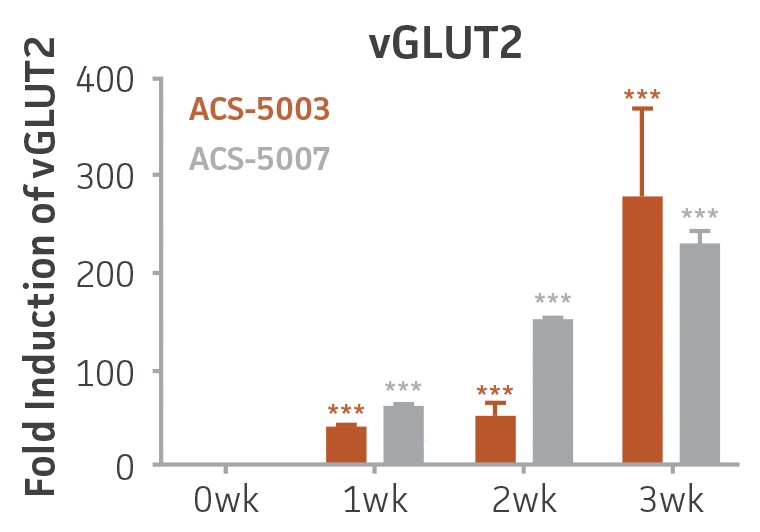
Figure 2. Induction of glutamatergic neuronal gene expression during 3 weeks of dopaminergic neuron differentiation. Expression of GLS2, vGLUT1, and vGLUT2 mRNA increased significantly in a time-dependent manner during 3 weeks (wk) of dopaminergic media treatment in ACS-5003 and ACS-5007 NPCs (n=1, *p < 0.05, **p < 0.01, ***p < 0.001 vs. day 0, Two-Way ANOVA).
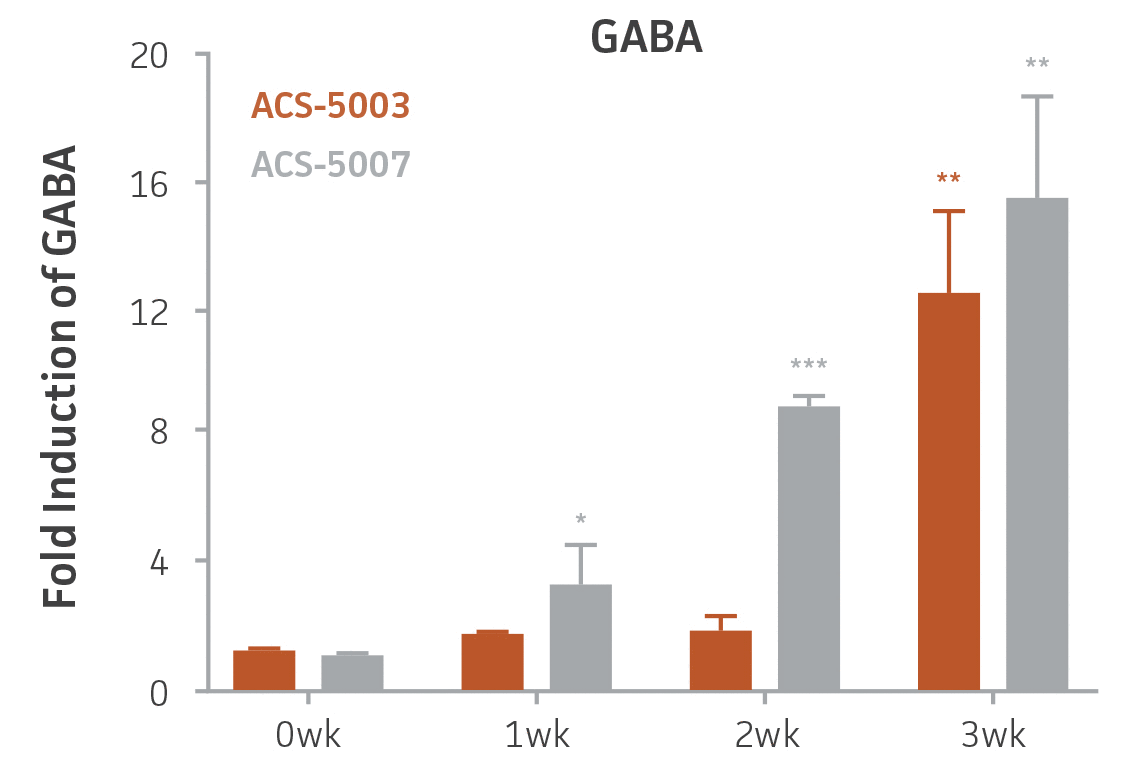
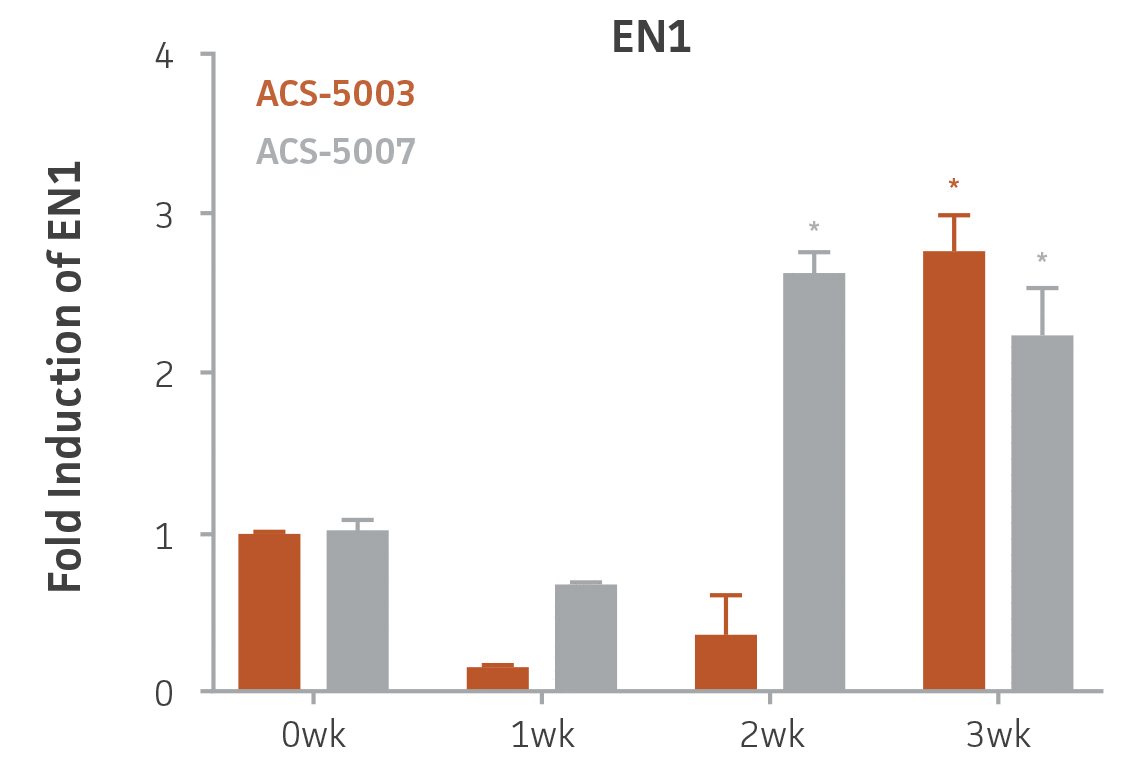
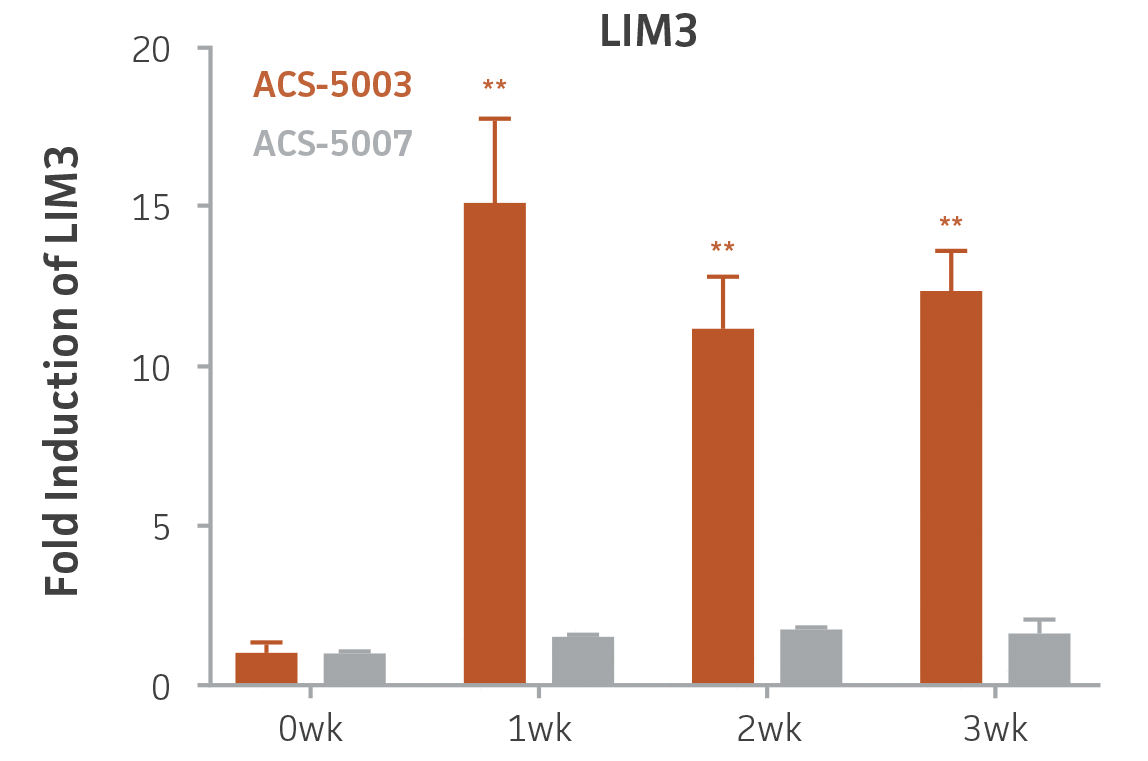
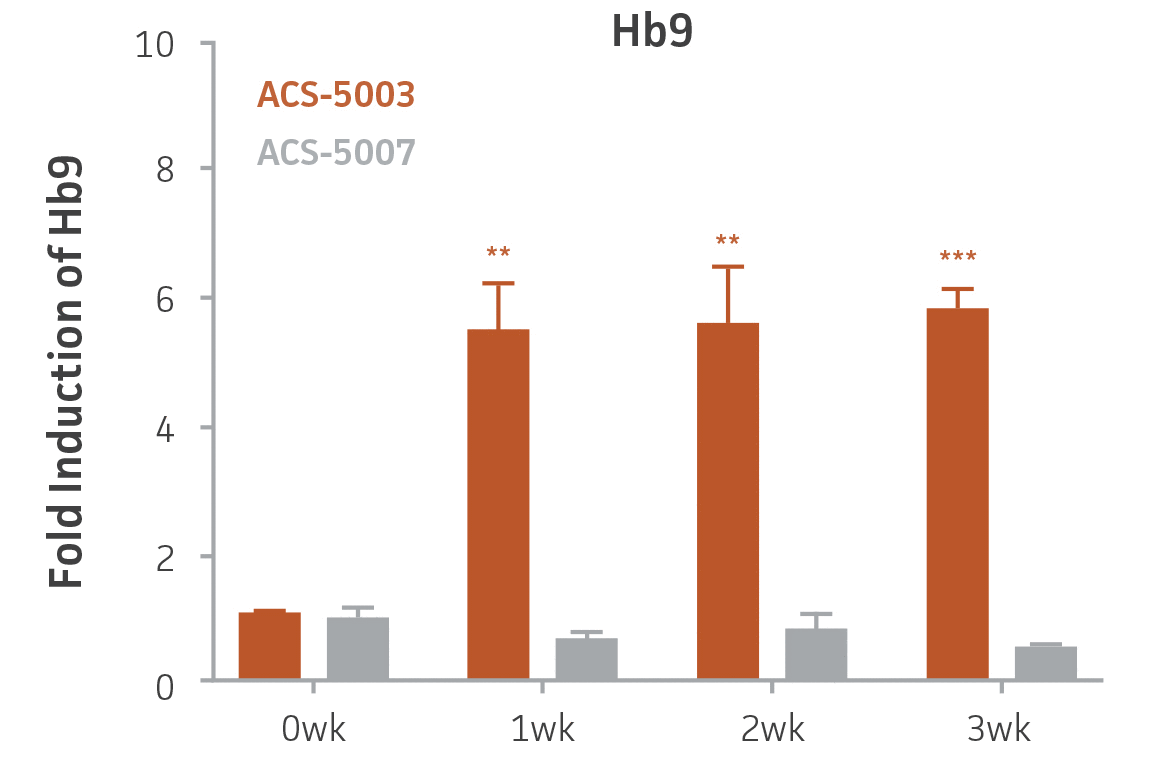
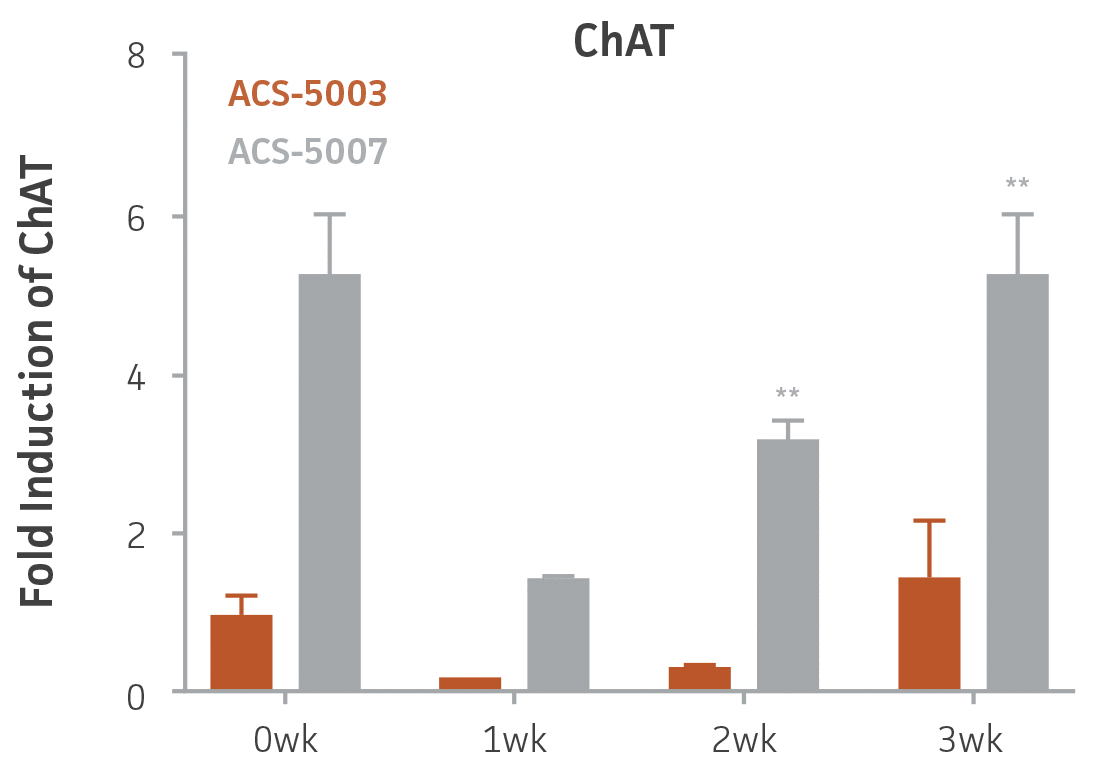
Figure 3. Induction of GABAergic, motor, and cholinergic neuronal gene expression during 3 weeks (wk) of dopaminergic neuron differentiation. GABA and EN1 mRNA increased significantly in both ACS-5003 and ACS-5007 NPCs in a time-dependent manner during neuronal differentiation. There was a significant increase in LIM3, and Hb9 mRNA in ACS-5003 while ChAT mRNA increased significantly in ACS-5007 NPCs during dopaminergic differentiation (n=1, *p < 0.05, **p < 0.01, ***p < 0.001 vs. day 0, Two-Way ANOVA).
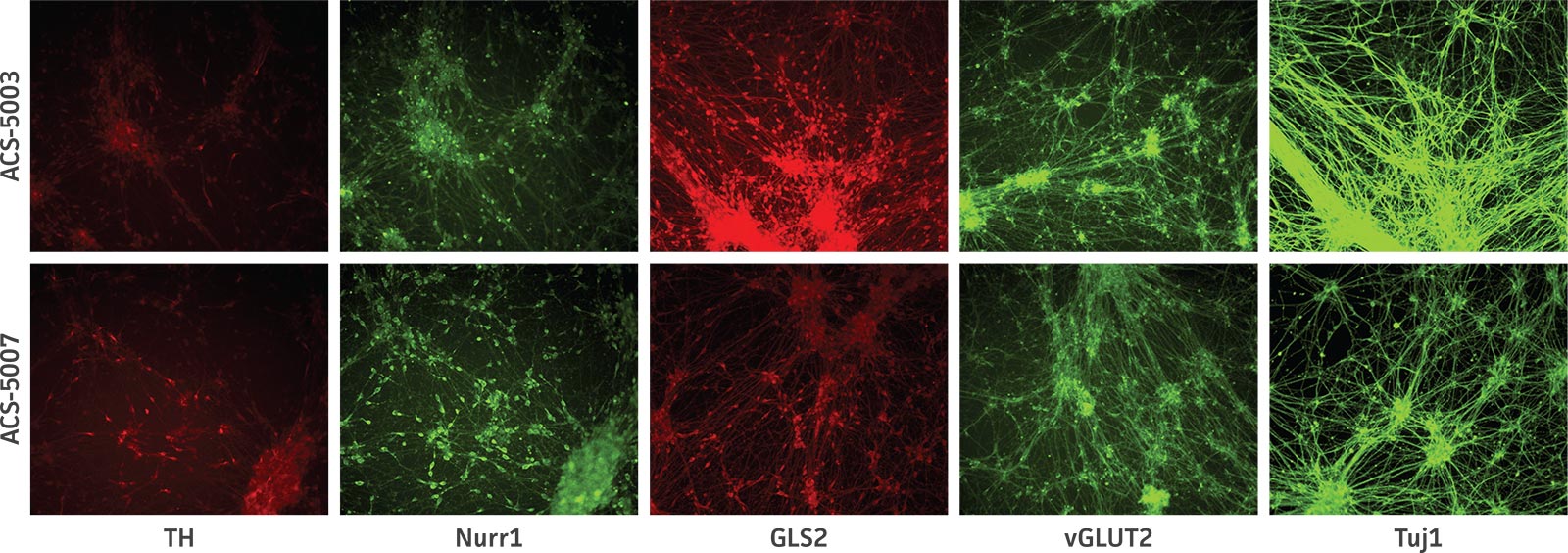
Figure 4. Immunocytochemistry of ACS-5003- and ACS-5007-derived neurons after 3 weeks of dopaminergic neuron differentiation. Consistent with qRT-PCR data, after 3 weeks incubation in dopaminergic differentiation media there was a marked increase in expression of TH, Nurr1, GLS2, vGLUT2, and Tuj1 neuronal markers. Original magnification, ×20.
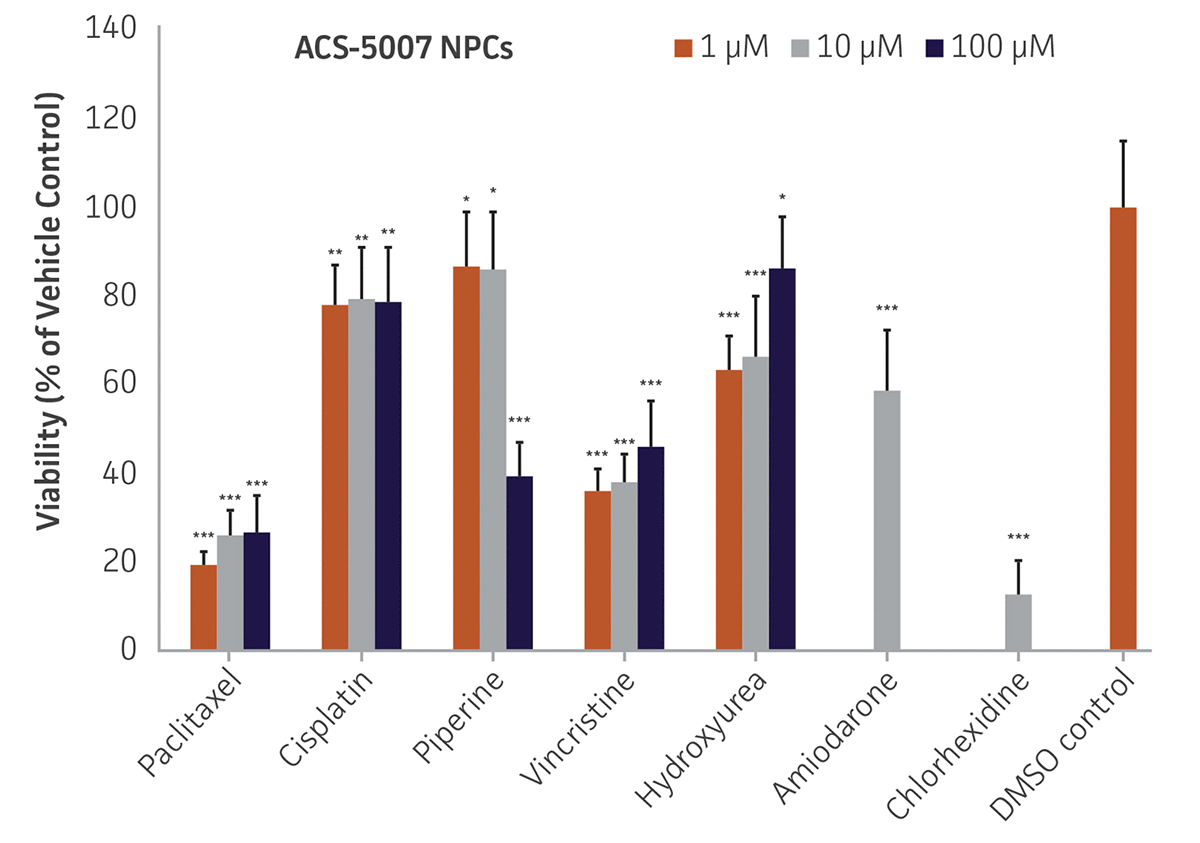
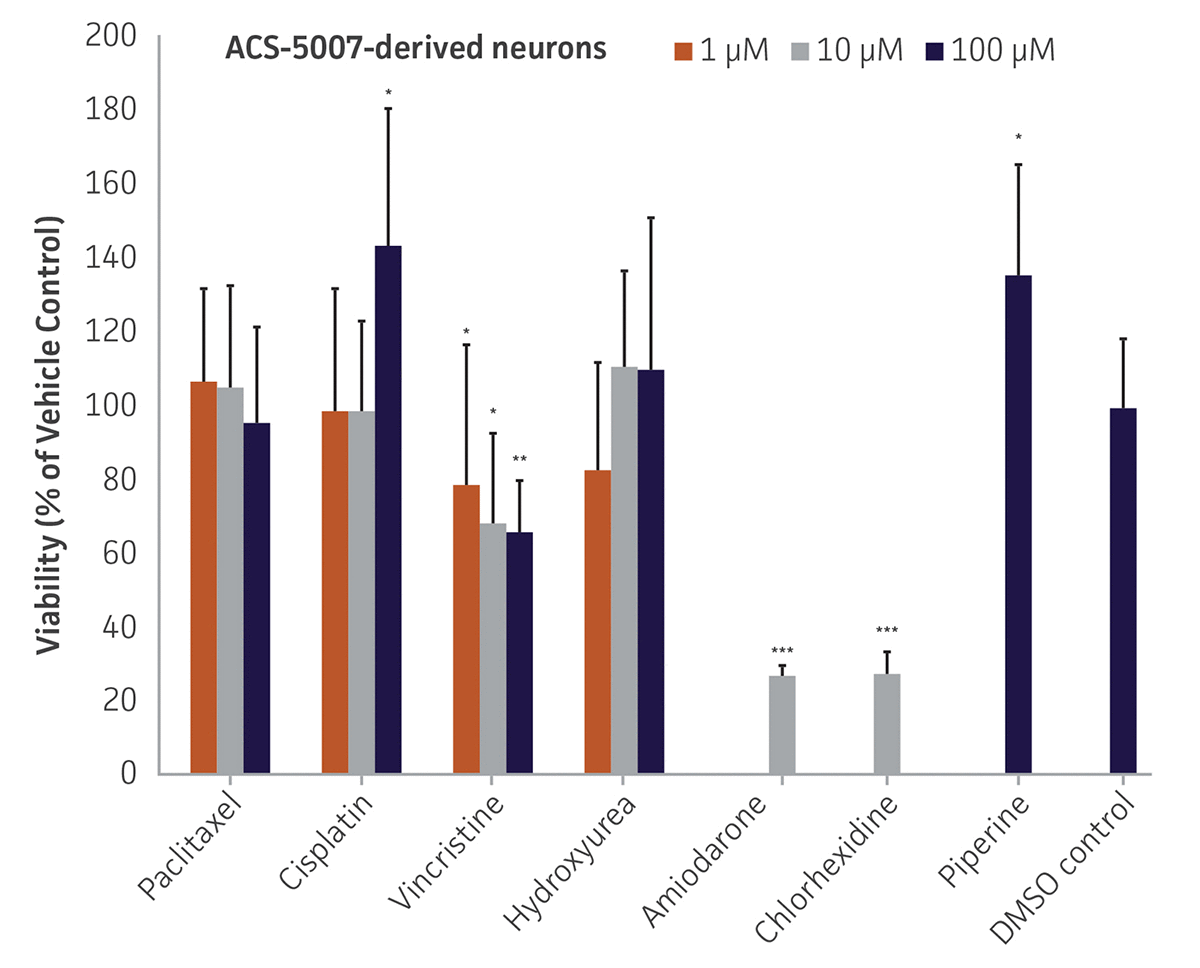
Figure 5. Dose-response curves for cell viability of ACS-5007 NPCs and ACS-5007 NPC-derived neurons treated with paclitaxel, cisplatin, piperine, vincristine, chlorhexidine, amiodarone, and hydroxyurea for 2 days. Paclitaxel, cisplatin, piperine, and hydroxyurea significantly induced neurotoxicity (p < 0.05) in NPCs but not in NPC-derived neurons. Vincristine, chlorhexidine, and amiodarone significantly decreased viability (p < 0.01) of both NPCs and neurons (n=3, *p < 0.05, **p < 0.01, ***p < 0.001 vs. DMSO control, Student’s T-test). The only concentration tested for amiodarone and chlorhexidine was 10 μM. Similar neurotoxicity was observed in ACS-5003 cells (data not shown).
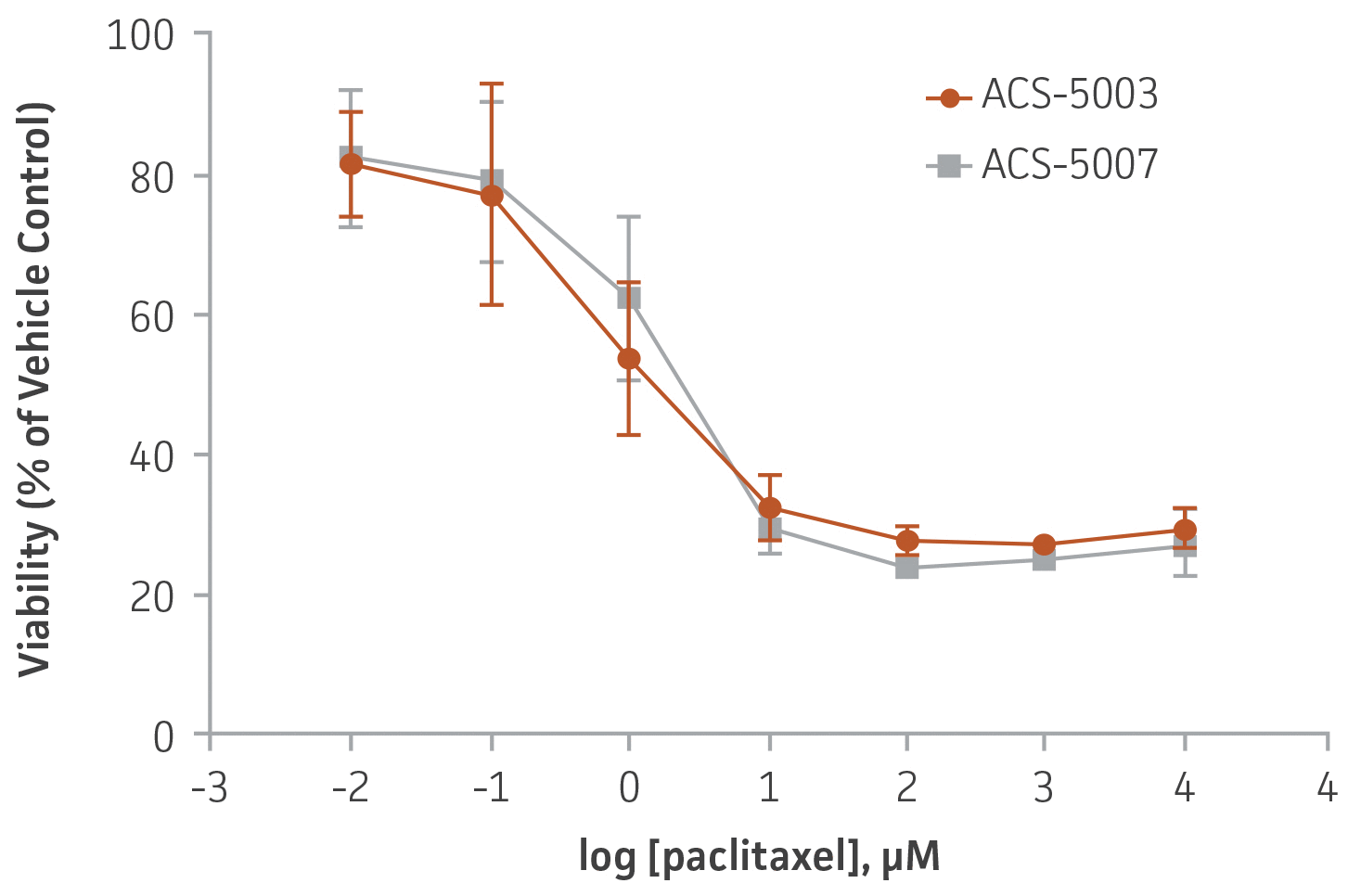
Figure 6. Dose-response curves for cell viability of ACS-5003 and ACS-5007 NPCs treated with paclitaxel for 2 days. The neurotoxic effect of paclitaxel was similar in both types of NPCs. IC50 of paclitaxel on ACS-5003 and ACS-5007 NPCs was 0.9 μM and 1.6 μM, respectively (n=2).
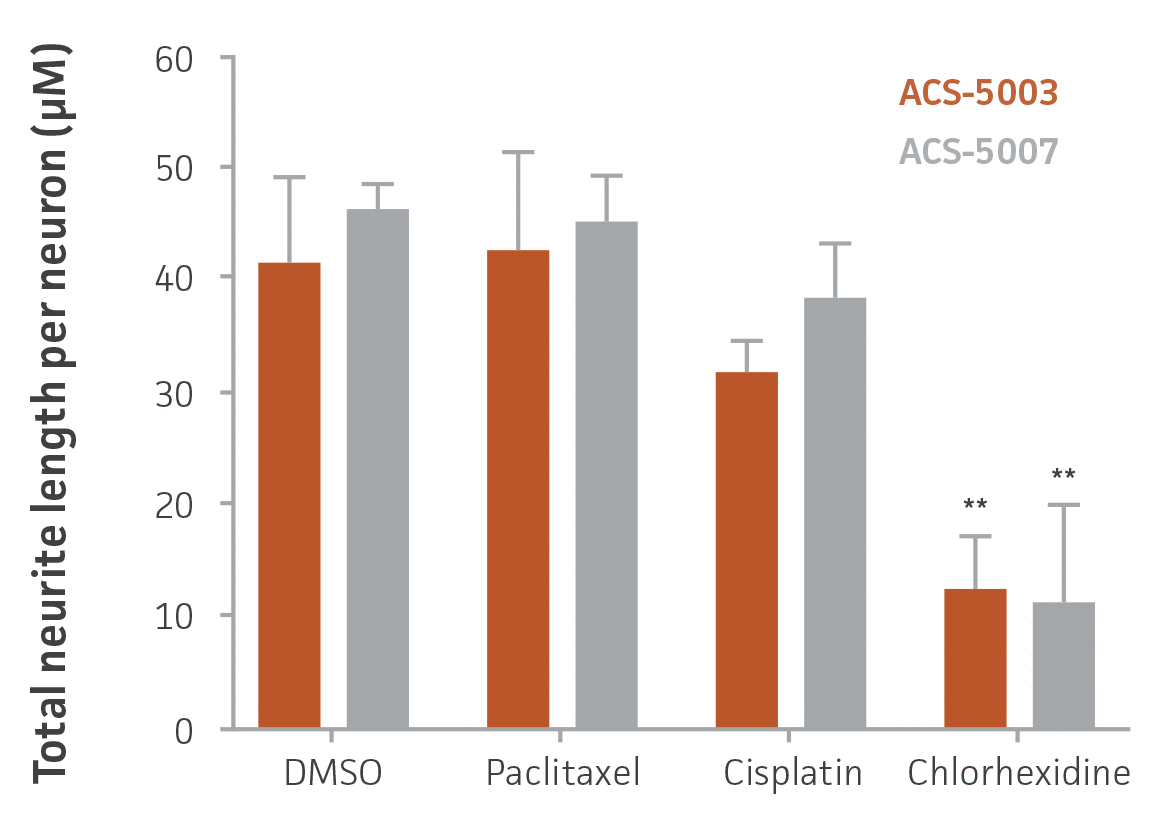
Figure 7. High-content imaging of chemotherapeutic-treated NPCs supports cell viability experiments. ACS-5003 and ACS-5007 NPCs-derived neurons were incubated with 10 μM paclitaxel, cisplatin, or chlorhexidine for 2 days; stained with Calcein Green AM and Hoechst 33342; and then subjected to high-content imaging. Consistent with the viability data, chlorhexidine significantly inhibited the neurite outgrowth (n=2, **p < 0.01 vs. DMSO, Student’s T-test). Original magnification, ×20.
Download a PDF of this application note
Download NowReferences
- Lopes C, Rego AC. Revisiting Mitochondrial Function and Metabolism in Pluripotent Stem Cells: Where Do We Stand in Neurological Diseases? Mol Neurobiol. DOI: 10.1007/s12035-016-9714-8, 2016. PubMed: 26892627
- Srikanth P, et al. Stem cells on the brain: modeling neurodevelopmental and neurodegenerative diseases using human induced pluripotent stem cells. J Neurogenet 28(1-2):5-29, 2014. PubMed: 24628482
- Schwartz MP, et al. Human pluripotent stem cell-derived neural constructs for predicting neural toxicity. PNAS 112(40):12516-21, 2015. PubMed: 26392547
- Csöbönyeiová M, et al. Toxicity testing and drug screening using iPSC-derived hepatocytes, cardiomyocytes, and neural cells. Can J Physiol Pharmacol 94(7):687-94, 2016. PubMed: 27128322
- Schmidt BZ, et al. In vitro acute and developmental neurotoxicity screening: an overview of cellular platforms and high-throughput technical possibilities. Arch Toxicol 2016 Aug 4. [Epub ahead of print]. PubMed: 27492622
- Shinde V, et al. Human Pluripotent Stem Cell Based Developmental Toxicity Assays for Chemical Safety Screening and Systems Biology Data Generation. J Vis Exp 7(100):e52333. doi: 10.3791/ 52333, 2015. PubMed: 26132533
- Westerink RH, Ewing AG. The PC12 cell as model for neurosecretion. Acta Physiol 192(2): 273-85, 2008. PubMed: 18005394
- Xie HR, et al. SH-SY5Y human neuroblastoma cell line: In vitro cell model of dopaminergic neurons in Parkinson’s disease. Chin Med J 123(8): 1086-92, 2010. PubMed: 20497720
- Cundiff PE, Anderson SA. Impact of induced pluripotent stem cells on the study of central nervous system disease. Curr Opin Genet Dev 21(3): 354-61, 2011. PubMed: 21277194
- Armstrong DL, The hippocampal tissue slice in animal models of CNS disorders. Neurosci Biobehav Rev 15(1): 79-83, 1991. PubMed: 2052203
- Pena F. Organotypic cultures as tool to test long-term effects of chemicals on the nervous system. Curr Med Chem 17(10): 987-1001, 2010. PubMed: 20156165
- Ledeen RW, et al. Gangliosides as neurotrophic agents: Studies on the mechanism of action. Acta Neurobiol Exp 50(4-5): 439-49, 1990. PubMed: 2130660
- Liu G, et al. Use of induced pluripotent stem cell derived neurons engineered to express BDNF for modulation of stressor related pathology. Front Cell Neurosci 8: 316, 2014. PubMed: 25352778