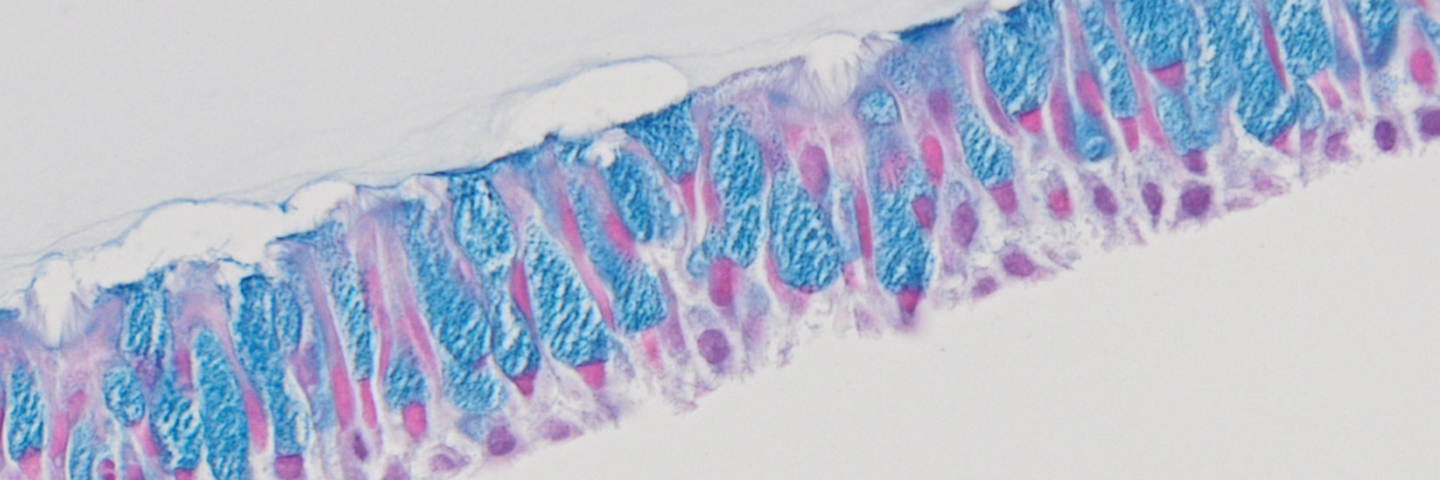
Author: Kevin Tyo, PhD
This report showcases an optimal method of fabricating airway models consisting of human bronchial tracheal epithelial cells (HBECs) grown in collagen coated 24-well plate inserts and cultured under air-liquid interface (ALI).
Introduction
Materials and Methods
Results
Discussion
References
Abstract
Human respiratory research encompasses a variety of fields including drug development, disease research, and toxicological testing. Despite the availability of traditional in vitro airway models, there is a lack of physiological relevance to the human lung. Advanced in vitro airway models have been previously generated that promise to provide more relevant results for use in respiratory research; however, the numerous variables associated in fabrication of these advanced models can cause incomplete or inconsistent differentiation, resulting in research delays or cost overruns. This report showcases an optimal method of fabricating airway models consisting of human bronchial tracheal epithelial cells (HBECs) grown in collagen coated 24-well plate inserts and cultured under air-liquid interface (ALI).
Models generated using different lots of primary HBECs and hTERT-immortalized HBECs were compared. In addition, various commercial medias designed to promote epithelial differentiation were also evaluated. Next, primary HBECs from both ATCC and other commercial primary cells were compared on epithelial differentiation and model morphology using optimized processes validated during the first phase of study. All airway models were evaluated via weekly microscopy and transepithelial/transendothelial electrical resistivity measurements. Additionally, H&E and alcian blue imaging and as well as MUC5AC and α-tubulin immunohistochemical (IHC) analysis from histological samples of mature models were generated. These studies elucidate techniques and procedures to reliably generate 3-D airway models with consistent full epithelial differentiation using both ATCC and other commercial primary HBECs.
Download a PDF of this application note
DownloadIntroduction
The human respiratory tract facilitates the vital function of air exchange within the human body. The bronchial tracheal tract, a subset of the lower respiratory tract, assists in performing this role by helping protect against pathological microorganisms common in the air and is an important focus in disease research. The bronchial tracheal tract is also commonly studied in both toxicology and pharmaceutical research, due to inhalation being a common route of toxicity as well as acting as a viable route of therapeutic drug administration respectively.1,2 The bronchial tracheal tract itself is characterized as possessing a pseudo-stratified columnar epithelium, comprised of goblet, ciliated, and basal cells. Here, goblet cells excrete mucin proteins entrapping microparticles and microorganisms, while ciliated cells serve to remove these particulates out of the respiratory tract.3
In recent decades, advanced in vitro models have been fabricated that better emulate the physiological complexity of the bronchial tracheal tract. In these models, undifferentiated HBECs are initially seeded on microporous membrane supports. By partially exposing these cells to the air, known as air-liquid interface (ALI), and incubating cells in specialized media for a period of 3-4 weeks, the HBECs will fully differentiate.4 Once fully differentiated, these models provide a physiologically relevant representation of the bronchial tracheal tract, mimicking both its’ morphology and composition. In contrast, traditional in vitro 2-D-airway models, typically comprised of just undifferentiated HBECs monolayers submerged in media, are limited in their ability to offer physiologically relevant data.5 In addition to providing physiologically relevant data, 3-D airway models can be easily fabricated, and offers researchers an affordable do-it-yourself model.
Another useful tool for researchers in the respiratory tract is the usage of human telomerase reverse transcriptase (hTERT)–immortalized primary cells.6 Here, primary cells are genetically modified to have exogenous expression of hTERT, conferring the ability to bypass cellular senescence. Unlike traditional primary cell lines, which have limited proliferation capability and are typically unable to form mature airway models after five cell passages, hTERT-immortalized cell lines have the potential for continuous proliferation and usage. The near unlimited proliferative capability of hTERT-immortalized cells also eliminates lot-to-lot variation from usage of multiple primary cell lots. Compared to previous immortalization cell lines, hTERT-immortalized cells possess near similar physiological and morphological properties as well as comparable growth rate relative to primary cells. A large variety of hTERT-immortalized cells have been created that are relevant to the respiratory tract, including the bronchial tracheal tract, offering a viable alternative to the usage of primary cell in respiratory research.
In the previous work “ATCC Human Bronchial/Tracheal Epithelial Cells: Improving Functional Studies”7 ATCC investigated and validated the ability of one lot of in-house primary HBECs to create in vitro 3-D airway models for use in respiratory studies. In these studies, ATCC further investigated the fabrication of 3-D airway models using primary HBECs incubated in collagen coated 24-well plate inserts under ALI. Here the epithelial differentiation potential of four separate lots of primary HBECs as well as hTERT-immortalized HBECs were compared. Additionally, factors that affect epithelial differentiation and replicate variability in airway models, such as plate layout and configuration were investigated. Different commercial ALI differentiation media and their effects on model maturation were evaluated as well. Next, subsequent studies were conducted to compare ATCCs primary HBECs with other commercial primary cells available for purchase. Here models generated using either primary cells from ATCC or from three other commercial vendors using optimized processes validated during the first phase of studies were compared on epithelial differentiation and model morphology.
These studies elucidate techniques and procedures that can be used to reliably generate 3-D airway models with consistent and full epithelial physiology and functionality using ATCC’s primary HBECs. In addition, this work shows that primary HBECs from ATCC can form functional airway models with similar competence as other commercially available primary cells.
Materials and methods
Initial cell culture
All four lots of Primary Bronchial/Tracheal Epithelial Cells; Normal, Human (ATCC PCS-300-010) and hTERT-immortalized NuLi-1 (ATCC CRL-4011) HBECs were grown according to their respective ATCC product sheet recommendations.8,9 Briefly, all cells were first grown in ATCC complete airway epithelial growth media, consisting of ATCC Airway Epithelial Cell Basal Medium (ATCC PCS-300-030) supplemented with Bronchial Epithelial Cell Growth Kit (ATCC PCS-300-040) and 5 mL Penicillin-Streptomycin solution (ATCC 30-2300). During culturing and cell proliferation, the cell passage number for all cell lines did not exceed two passages. Cell growth media was replaced every other day.
One day prior to cell seeding, permeable trans-well inserts for 24-well plates with polyester (PET) membrane with 0.4 μm pores (Corning cat# 353095) were placed into Costar clear 24-well multi-well plates (Corning cat# 3524). To assess possible edge effects on airway models, inserts were either added to each well, or to interior wells only. The plate inserts were then coated on the apical side with 0.3 mg/mL Collagen solution (Stemcell Technologies cat# 04902) diluted with Dulbecco’s Phosphate Buffered Saline (DPBS) (ATCC 30-2200), with plates incubated overnight at 4°C.
On the day of seeding, the collagen-coated inserts were apically rinsed twice with 200 μL DPBS, with 0.5 mL of ATCC complete airway epithelial growth media added to the basal side of wells containing trans-well inserts. Wells without inserts were filled with 2 mL DPBS. HBECs were passaged, harvested, and then resuspended in growth media at a concentration of 500,000 cells/mL. The cell solution was added to each insert at a volume of 0.2 mL (100,000 cells per well). Following cell seeding, plates were left at room temperature for at least one hour to allow for cell attachment, followed by placement in 37°C incubation. Cells were incubated for 2-3 days until full confluency was reached. On the second day of incubation, both apical and basal side media was replaced with 0.2 and 0.5 mL of complete growth media respectively.
In the subsequent studies, two lots from different commercial HBECs were cultured in their respective growth medias and were grown under equivalent conditions to ATCC HBECs. All cells underwent the same cell seeding procedure, except for the usage of the respective growth media. All plates used in comparing ATCC and commercial HBECs contained trans-well inserts in interior wells only.
ALI culturing
Once reaching full confluency, both the apical and basal media were removed from each airway model. In the first set of studies (Table 1), the basal media was replaced with 0.5 mL of either 1) ATCC complete airway epithelial growth media, 2) mixture of (80%) ATCC complete airway epithelial growth media supplemented with (20%) complete fibroblast media (ATCC PCS-201-030 supplemented with ATCC PCS-201-041, and ATCC 30-2300), 3) Air-Liquid Interface Epithelial Differentiation Medium (Lifeline Cell Technologies cat# LM-050), or 4) PneumaCult ALI maintenance media (Stemcell Technologies cat# 05001) supplemented with appropriate components (Stemcell Technologies cat# 07980 and 07925). In subsequent studies, basal media was replaced with 0.5 mL of either PneumaCult ALI maintenance media or respective competitor ALI differentiation media (Table 2). For all studies, basal media was replaced every other day. Following 2 weeks of ALI incubation, weekly apical washes with (2x) 0.2 mL DPBS were conducted to remove excess mucin from models. Weekly trans-epithelial electrical resistance (TEER) measurements were conducted as well. After 5 weeks of ALI incubation, models were considered mature and further processed.
Differentiation studies
During ALI incubation, weekly microscopy images from all samples were collected using EVOS FL digital microscope (Thermo Fisher Scientific). Tight junction formation was observed via weekly TEER measurements following 2 weeks of ALI incubation and were collected using EVOM2 Epithelial voltmeter (World Precision Instruments). Tight junctions were also assessed via FITC-Dextran transmembrane permeability. Briefly, 100 μL of 1 mg/mL of 4,000 MW FITC-Dextran (Sigma Aldrich) in DPBS was added apically to airway models acclimated at room temperature. Aliquots of basal media were taken at 4 hours following dextran administration, and were measured via fluorescence (ex. 490 nm, em. 520 nm).
Following ALI incubation, selected airway model replicates were fixated and permeabilized using BD cytofix/cytoperm fixation and permeabilization solution (BD cat# BD 554722). Samples were stored in 10% ethanol at 4 ˚C until further processing. Preserved models were paraffin embedded, sectioned, and stained with either H&E or alcian blue, followed by histological examination and imaging. Additional paraffin embedded samples underwent IHC staining and imaging using both α-tubulin (Invitrogen cat# 32258) and MUC5AC (Cell Signaling Technology cat# 6119) antibodies.
Results
Weekly microscopy images were collected for all airway models throughout the ALI incubation period. During the initial week of ALI incubation, little to no differences between airway models were observed (Figure 1A-B). However, during the second week of ALI, morphological differences between airway models incubated in different medias became apparent. These morphological differences between media choice became more pronounced as the weeks progressed, culminating in diverse model morphologies following 5 weeks of ALI (Figure 1C-F). Models incubated in bronchial growth media exhibited bare spots on the membrane at 3 weeks, which increased in size as the weeks progressed. In contrast, cells incubated in the other media showed no decrease in cellular confluency. Model morphology was affected only by choice of differentiation media, whereas little to no lot-to-lot variation between primary HBECs was observed. However, models comprising hTERT-immortalized NuLI-1 bronchial cells demonstrated higher cellular density morphology compared to primary cell counterparts (data not shown).
Weekly TEER measurements were also conducted to assess tight junction formation. Although lot-to-lot variation from primary HBECs was observed, again, differentiation media choice played a larger role in affecting TEER values (Figure 2A). Models using bronchial epithelial growth media exhibited minimal resistivity, relative to other medias. Moreover, hTERT-immortalized NuLI-1 model controls also demonstrated minimal resistivity, regardless of media choice. The edge effect was also found to contribute to TEER values, with increased TEER variability observed in airway models grown in full 24-well plates compared to airway models incubated inner wells only (Figure 2B). Finally, histology assessment was conducted on H&E and alcian blue stained paraffin embedded airway models. Representative images of airway models are shown in (Figure 3). Models comprised of primary HBECs incubated only with complete bronchial growth media demonstrated no epithelial differentiation, as well as low cellular confluency, probably due to contact inhibition. In contrast, models incubated in 80:20 mixture of bronchial: fibroblast growth media showed full model confluency, but minimal epithelial differentiation. Finally, models grown in either Lifeline Technology’s or Stemcell Technology’s ALI medias demonstrated proper model morphology as well as appropriate epithelial differentiation, with both goblet cells and ciliated cells present. It was observed that the use of Stemcell Technology’s ALI media provided models with increased model thickness, relative to other media. Finally, histological imaging of hTERT-immortalized NuLI-1 controls cultured in either ALI media showed that hTERT cell models are unable to form mature airway models with physiologically relevant morphology or appropriate epithelial differentiation.
After the initial study, all subsequent models were incubated in Stemcell media using only interior wells in 24-well plates. In the following study, airway models comprising of ATCC primary HBECs were compared with models formed from commercial vendors’ primary cells using either Stemcell media or their respective commercial media (Table 2).
Weekly TEER measurements were conducted in airway models. (Figure 4) shows the total resistivity of all tested airway models in either (A) Stemcell Technologies media or (B) respective commercial differentiation media. Although differences between lots and media choice was present, resistivity values for all models were acceptable. The only exception was Lot 1 from the 1st commercial vendor, which showed low TEER values both in Stemcell Technology media and the respective commercial differentiation media, indicating suboptimal tight junction and model formation.
Following ALI incubation, functional studies on model tight junction formation and mucin production were assessed via FITC-dextran kinetics and MUC5AC ELISA respectively (Figure 5). FITC-dextran transmembrane diffusion analysis on selected models revealed that the rate of dextran diffusion was inversely proportional to TEER values, and that decreased dextran basal concentrations were relative to increased model TEER values (Figure 5A). All selected airway models showed the presence of MUC5AC, demonstrating epithelial differentiation with the presence of functional goblet cells (Figure 5B).
Epithelial differentiation was confirmed via histological analysis (Figure 6). Here representative images from alcian blue stained airway models incubated in Stemcell Technology ALI media are shown. all airway models demonstrated acceptable model integrity with appropriate epithelial differentiation, showing the presence of both goblet and ciliated cells, with the exception of lot 1 from commercial vendor 1 (Figure 6D). Moreover, models matured in Stemcell Technology ALI media demonstrated higher epithelial thickness, relative to models grown in Lifeline Technology ALI media (data not shown).
Finally, IHC staining for α-tubulin and MUC5AC was conducted on selected airway models. Representative images from IHC staining are shown in (Figure 7), with all selected samples demonstrating the presence of both for α-tubulin and MUC5AC, proteins that are only expressed on fully functional and differentiated bronchial epithelial cells.
Morphology was consistent between different HBEC lots in the same media
Figure 1. Morphology of airway models is dependent upon ALI media.Microscopy images representative of HBECs (A) prior to ALI culturing, (B) all models after one week of ALI, as well as 5 week model incubation using (C) complete bronchial growth media, (D) 80:20 bronchial: fibroblast growth media, (E) Lifeline Technology ALI media or (F) Stemcell Technology ALI media. Little to no morphology differences were shown between different primary HBECs lots incubated in the same differentiation media (data not shown). Scale bar represents 400 μm.
Tight junction formation was sensitive to the edge effect
Figure 2: Comparing TEER differences in airway models grown in different differentiation media as well as assessing edge effect. (A) Total resistivity measurements from airway models incubated using different differentiation media. (B) Comparisons of percent error between airway models using all wells within a 24-well plate and models incubated in the interior wells only.
Histology assessment revealed model-dependent differences in differentiation
Figure 3: Histological assessment of H&E and alcian blue stained paraffin embedded airway models. Representative alcian-blue stained histological images of airway models comprised of primary HBECs were incubated with (A) complete bronchial growth media, (B) 80:20 bronchial: fibroblast growth media, (C) Lifeline Technology, or (D) Stemcell Technology ALI media. Images of airway models comprised of hTERT-immortalized cell NuLI-1 controls cultured in either (E) Lifeline Technology or (F) Stemcell Technology ALI media. Scale bars represent 20 μm.
Weekly TEER measurements for all models were within the acceptable range
Figure 4: Resistivity of all tested airway models. TEER values from airway models comprised of primary HBECs from either ATCC or other commercial vendors incubated in either (A) Stemcell Technology or (B) respective commercial ALI differentiation media.
Functional studies indicated tight junction formation and mucin production
Figure 5: Functional studies on selected airway models. (A) Tight junctions were evaluating by comparing the rate of transmembrane diffusion of FITC-Dextran in selected airway models. (B) Mucin production (MUC5AC), an indicator of epithelial differentiation, was evaluated in selected airway models via ELISA.
Epithelial thickness varied between models
Figure 6: Confirmation of epithelial differentiation. Epithelial differentiation was confirmed via histological analysis. Representative alcian-blue stained histological images of airway models incubated in Stemcell Technology ALI media comprised of primary HBECs from (A) ATCC lot 1, (B) ATCC lot 2, (C) ATCC lot 3, (D) Commercial Vendor 1 (Lot 1), (E) Commercial Vendor 1 (Lot 2), (F) Commercial Vendor 2 (Lot 1), (G) Commercial Vendor 2 (Lot 2), or (H) Commercial Vendor 3 (Lot 1). Scale bars represent 20 μm.
IHC staining demonstrated the presence of both α-tubulin and MUC5AC
Figure 7: IHC staining for α-tubulin and MUC5AC. Representative images from IHC stained airway models from (A) ATCC lot 1, (B) ATCC lot 2, (C) ATCC lot 3, and (D) Commercial vendor 3 (Lot 1). Red fluorescence in images is from MUC5AC, green fluorescence stems from α-tubulin, with DAPI control as blue.
Discussion
In this report we set out to identify an optimal method of fabricating airway models consisting of HBECs cultured under ALI. In the first set of studies, different medias and blends were evaluated to discover the most optimal solution to reliably induce epithelial differentiation in airway models. Additionally, the configuration of trans-well positioning in 24-well plates to minimize the edge effect and reduce replicate variability was also investigated. Here we demonstrated that using either Stemcell Technologies PneumaCult ALI media or Lifeline Technology ALI Epithelial Differentiation media provided optimal epithelial differentiation and airway model thickness, relative to other tested medias. Additionally, by adding inserts to the inner wells only (as well as adding DPBS to the outer wells), replicate variability from the edge effect is minimized. When combining optimal trans-well configuration with an appropriate ALI differentiation media, more consist replicate data is generated. Finally, this work, in line with previous studies, demonstrate that hTERT-immortalized HBECs are unable to form physiologically relevant 3-D airway models, compared to the usage of primary cells.10
In the second set of studies, various commercial primary HBECs were compared to ATCC primary HBECs. Using the optimized protocols elucidated in the first set of studies, airway models were generated using either Stemcell Technologies PneumaCult ALI or respective commercial ALI differentiation media. Here, airway models comprising either ATCC or commercial vendor cell lines were successfully generated. These results demonstrate that ATCC primary HBECs are an effective tool to generate airway models with appropriate epithelial differentiation, model morphology, and mature functionality and can be incorporated into advanced toxicological studies.
Acknowledgement
Histological processing and imaging were conducted by VitroVivo Biotech LLC.
Download a PDF of this application note
DownloadTable 1: Media Differentiation Test
Layout of first set of studies comparing different media during ALI incubation. Four different primary cell lots were tested as well as NuLI-1 hTERT-immortalized primary cells (ATCC CRL-4011). Primary Bronchial/Tracheal Epithelial Cells; Normal, Human (ATCC PCS-300-010).
Table 2: Primary Cell Comparison
Layout of second set of studies comparing differentiation potential of primary cells from both ATCC and commercial vendors.
References
- Upadhyay, S. and L. Palmberg, Air-Liquid Interface: Relevant In Vitro Models for Investigating Air Pollutant-Induced Pulmonary Toxicity. Toxicological Sciences, 2018. 164(1): p. 21-30.
- Steimer, A., E. Haltner, and C.M. Lehr, Cell culture models of the respiratory tract relevant to pulmonary drug delivery. J Aerosol Med, 2005. 18(2): p. 137-82.
- Cao, X., et al., Invited review: human air-liquid-interface organotypic airway tissue models derived from primary tracheobronchial epithelial cells-overview and perspectives. In Vitro Cell Dev Biol Anim, 2021. 57(2): p. 104-132.
- Rayner, R.E., et al., Optimization of Normal Human Bronchial Epithelial (NHBE) Cell 3-D Cultures for in vitro Lung Model Studies. Sci Rep, 2019. 9(1): p. 500.
- Bérubé, K., et al., Human primary bronchial lung cell constructs: the new respiratory models. Toxicology, 2010. 278(3): p. 311-8.
- Poole, J.C., L.G. Andrews, and T.O. Tollefsbol, Activity, function, and gene regulation of the catalytic subunit of telomerase (hTERT). Gene, 2001. 269(1): p. 1-12.
- Aaron Briley, B.C.Z., PhD; and Brian Shapiro, PhD. ATCC Human Bronchial/Tracheal Epithelial Cells: Improving Functional Studies. Available from: https://www.atcc.org/resources/application-notes/atcc-human-bronchial-tracheal-epithelial-cells.
- ATCC, Primary Bronchial/Tracheal Epithelial Cells; Normal, Human PCS-300-010. Product Information Sheet.
- ATCC, hTERT-immortalized Human cells NuLi-1 CRL-4011. Product Information Sheet.
- Wang, H., et al., Establishment and comparison of air-liquid interface culture systems for primary and immortalized swine tracheal epithelial cells. BMC Cell Biol, 2018. 19(1): p. 10.